Jon Chorover, Department Head, Environmental Science, and a researcher with The University of Arizona’s Superfund Research Center, has been exploring the health impacts of hazardous waste created by mining operations in the southwestern US. An expert in soil systems, Chorover is applying his extensive experience to study the potential use of biochar to remove toxic compounds from acid mine drainage (AMD).
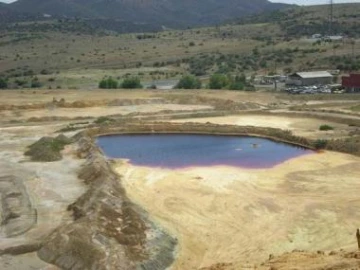
Acid mine drainage at the Iron King Mine Humboldt Smelter Superfund Site
“Mining is obviously a very important activity here. It generates a lot of precious metals that are utilized in various aspects of our economy, but it also generates a very large quantity of earth material waste that is mined from well below the surface,” Chorover says. After materials are brought up to the surface and the precious metals are extracted, the leftover waste material is deposited on the landscape. It’s not uncommon for mining waste to contain sulfide minerals like arsenopyrite and galena, which are stable underground, but weather and transform quickly when exposed to oxygen and water from the local climate. The oxidization process releases toxic compounds like arsenic and lead, making them more biologically available in the environment, or much more likely to be absorbed by humans and other organisms.
“These are very fine particles that form,” Chorover says. “We’re concerned with the extent to which wind and water may transport these fine particles into adjacent communities and impact the exposure to these very toxic elements.” For example, drainage water that passes through tailings on the landscape can absorb the released toxic elements—like arsenic and lead—and transport them directly into groundwater or surface water supplies. This then creates a big risk for the communities using those water sources. “There’s no way to transform arsenic into something else that isn’t arsenic,” Chorover says. “Once it’s in something, it’s a hazardous material.” In searching for solutions, Chorover’s team evaluated biochar as a potential way to reduce the transport of a form of arsenic known as arsenate.
All about biochar
Biochar is similar to regular charcoal; is a commonly produced waste product of burning wood and vegetation. Previous studies found that when biochar was introduced to a solution of arsenic and water, nothing really happened. However, when it was placed in the path of AMD, it resulted in significant uptake of the arsenic from solution. Chorover’s goal was to answer the million-dollar question: why was it more effective?
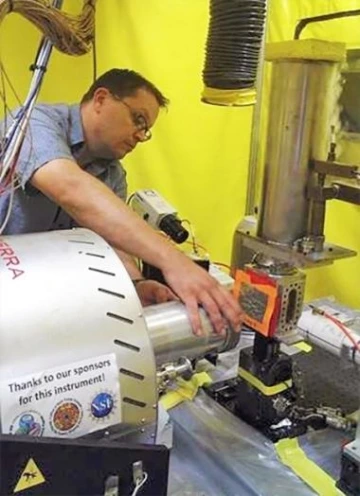
Dr. Robert Root, Associate Research Professor in Department of Environmental Science, conducting research at Stanford Synchrotron Radiation Lightsource (SSRL)
Thanks to the help of two synchrotron facilities (one in Canada and one in Stanford, CA), Chorover and his team were able to use high intensity x-rays to study the structure of and interactions between the biochar and the hodgepodge of chemicals in an AMD solution. These images revealed that the key factor wasn’t the biochar or the arsenic, but rather something else entirely. Ferric iron, also known as iron(III), forms when sulfide minerals oxidize, so it wasn’t surprising to find it in samples of AMD. According to Chorover, “this iron(III) is very attracted to certain types of chemical functional groups on the biochar and attaches quite strongly to those functional groups.”
As it turns out, the arsenic in solution was then forming incredibly strong covalent bonds onto the crystalized ferric iron coating, making a sort of sandwich. If the biochar and arsenic were slices of bread, then the iron was the meat in between. “What this result indicated to us was that the iron itself in acid mine drainage plays a very important role in functionalizing the surface of the biochar, making it more effective for uptake of the arsenic from solution,” Chorover says.
Managing hazardous materials
Eventually, there will be no more free spaces on the biochar for the iron(III) and subsequent arsenic to bind with, and the setup will need a fresh batch. The timeline for replacing the biochar varies greatly. Depending on the rate of water flow and concentrations of each compound, a biochar barrier could remain in place anywhere from a week to a few years. Then, the somewhat-stabilized toxic materials must be dealt with. “You have this arsenic-enriched biochar, which is effectively a hazardous waste and has to be disposed of as such. The benefit is that you’ve concentrated this arsenic into this form, so that it can be disposed of in a compact way,” Chorover says. “We’re able to remove it from potentially contaminating waterways and water supplies but ultimately, we would need to remove that carbon and dispose of it safely.”
Now that we know that biochar can be useful in absorbing arsenic from AMD, the next steps are to develop and test an upscaled version of the experiments in actual AMD sites. Chorover believes that mining and environmental engineers could work together to construct large-scale biochar permeable reactive barriers that would be able to test the chemical interactions in an actual field scenario. He would also love to explore a wider range of AMD chemistries to see when this system works and when it begins to break down.
“It is now recognized by many in the mining industry that there is a need to address the entire mining life cycle from exploration through remediation,” Chorover says. “The work we’re doing is addressing that tail end of the mining life cycle and developing an understanding of how that mine waste behaves in the human-populated environment.”
More information
Publication
Wang D, Root RA, Chorover J. Biochar-templated surface precipitation and inner-sphere complexation effectively removes arsenic from acid mine drainage. Environ Sci Pollut Res Int. 2021 Apr 18. doi: 10.1007/s11356-021-13869-8. https://doi.org/10.1007/s11356-021-13869-8